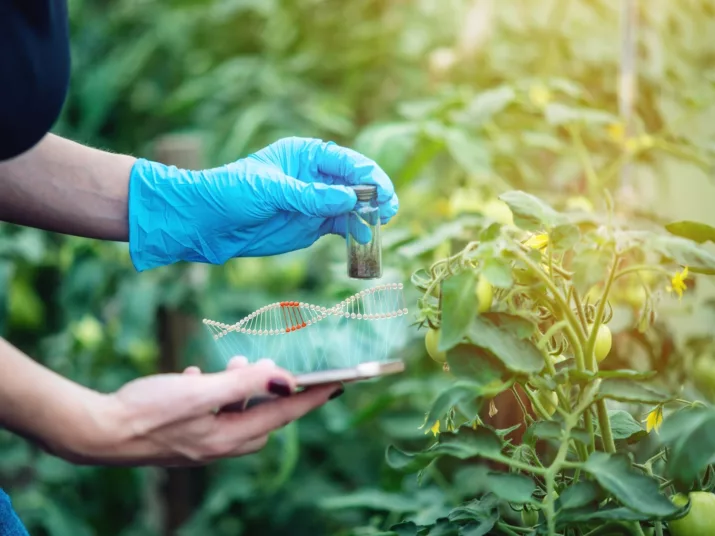
Photo: Artem / Fotolia
Thinking Space Nature
Creating Superplants with Gene Scissors
How can field crops be made to grow even faster and stronger? And what is the best way of combatting the pests that prey on them? For thousands of years, crop farmers around the globe have been asking themselves this sort of questions. One question in particular is becoming crucially important: how can we ensure that we’ll have enough food at our disposal in the future? According to United Nations’ estimates, world population is likely to grow to 9.7 billion people by 2050. Compared to today, this means around 2.5 billion additional people who will need to be fed.
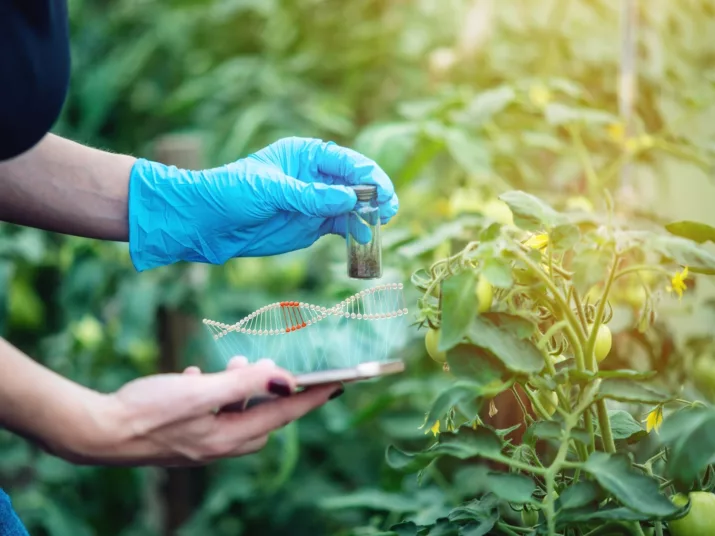
Photo: Artem / Fotolia
New plant breeding methods – above all genetic engineering – promise quick solutions: robust plants that in times of climate change are able to withstand extreme weather and diseases, while still guaranteeing high yields. In addition, these plants need to be able to fit in perfectly with sustainable agriculture to protect the environment and conserve resources.
Changing Genetic Material Instead of Crossbreeding to Produce Special Properties
A gold-rush mood is currently prevailing among scientists in the forefront of “genome editing”. For the journal MIT Technology Review, this new method is “the biggest biotech discovery of the century”. With its help, the genetic material of plants can be altered and rewritten. This makes redundant traditional and lengthy plant breeding procedures, in which plants with different properties are crossed with each other.
What’s so special about genome editing is that, unlike conventional genetic engineering methods, certain applications of the new method don’t require the use of genes that are foreign to the species. This is because the technology makes it possible to precisely modify the plant’s own genetic material with the help of gene scissors – for example, to make the plants resilient against pathogens.
Tools against Mildew and other Pathogens
In the case of mildew, a plant disease whereby fungi cover parts of the plant with a white coating and cause them to dry out, it works like this: the fungus penetrates the cells of wheat plants with a kind of mini tube to supply itself with nutrients. For this purpose, it requires a certain protein. This protein is located on the surface of a cell of the wheat plant. If the wheat cell lacks this protein, because it was previously removed from the plant’s genetic material by means of a gene scissors, the fungus can no longer harm the wheat.
Whether genome editing is used to make plants immune to diseases or to make fruits and cereal grains grow larger, the principle according to which the gene scissors works is always similar:
1. Precise search: Researchers apply genetic engineering methods to introduce special protein complexes from bacteria – so-called restriction enzymes (in the case of CRISPR/Cas9, the Cas9 “cutting protein”) – and a recognition molecule (the “guide RNA”) into the plant cells. In the cell, the guide RNA directs the Cas9 enzymes to search for and identify the exact spot within the plant genome where the “target DNA” is to be split.
2. Exact cut: Like a micro-scalpel, the enzymes cut through the plant DNA – exactly at the defined position.
3. New structures: The plant’s own repair enzymes try to repair the damage to the DNA. There are two repair mechanisms. Homologous recombination enables gene correction or the insertion of DNA segments at a specific sequence by means of an added template. This enables the plant to develop new properties. In non-homologous recombination, the two ends of the DNA are recombined without a template, whereby errors (mutations) may arise that damage the gene and render it inoperable. In this way, plants have been made resistant to the mildew pathogen. This was possible because the pathogen needs a specific protein (receptor) on the surface of the cell in order to enter it. If this receptor is destroyed, the pathogen can no longer enter and the plant is resistant. Gene inactivation is easier to perform by non-homologous recombination than by homologous recombination.
Milestones in Genome Editing:
1978
Werner Arber, Daniel Nathans and Hamilton Othanel Smith receive the Nobel Prize in Physiology or Medicine for their groundbreaking work on the “discovery of restriction enzymes and their application to problems of molecular genetics ”. Years later, the protein complex they were investigating – which among other things serves bacteria as a virus defence – is used to recognise and cut up specific parts of DNA.
1996
Artificially produced protein complexes, so-called zinc finger nucleases (ZFN), can for the first time cut DNA in a targeted manner. The process takes up to two months and costs between USD 1,000 and 25,000 (about EUR 900 to 22,500).
2010
Development of the TALEN (transcription activator-like effector nuclease) technology, which enables researchers to produce artificial protein complexes more easily and quickly – in less than a week.
2012
Development of the CRISPR-Cas technology (clustered regularly interspaced short palindromic repeats) – a method that makes genome editing easier, faster and more cost-effective than ever before. The method costs less than USD 100 (about EUR 90), and planning and implementation take no more than a few days. This is because the genetic scissors functions more precisely than previously and can be used in almost any kind of living cell and organism. It breaks new ground in fighting AIDS or cancer, but also in breeding plants and animals. First CRISPR-edited plants are tested in the field.
2012
Rapid increase in genome editing and thousands of publications on the subject. The method triggers a worldwide revolution in laboratories.
2015
Science Magazine confers the award “Breakthrough of the Year” to the CRISPR genome editing method.
2015
“Arctic apples” are approved for commercial launch without labelling in the USA: developed through a genetic engineering process (method: RNA interference), the apples have been modified in such a way that they don’t turn brown after being cut open and look fresh longer than non-modified apples. “Arctic apples” have been on the US market since 2017. Since 2016, gene-edited CRISPR mushrooms have been approved without labelling for the US market.
2018
The European Court of Justice classifies genome-editing processes as genetic engineering. Ever since, experts have been discussing whether this classification is appropriate. Another issue under discussion is whether the new methods could be compatible with organic farming.
Current Debate: Labelling Requirements
But what are the risks associated with genome editing and its range of applications? Will it have long-term effects on our health or the environment? The large number of unanswered questions nurtures consumers’ scepticism about genetic modification in breeding processes. Critics are currently calling for the compulsory labelling of genetically modified food. As a rule, even molecular biological analyses are unable to clearly determine whether genetic differences are the result of natural, that is, random mutations – or whether the latter are due to breeding processes such as genome editing.
Strict Guidelines in the EU
What’s allowed, and where, varies from country to country. If longer DNA sections – or even a complete gene – are incorporated into the plant genome, the plant is considered by international consensus to be a genetically modified organism (GMO) and its use is regulated accordingly. Within the EU, requirements are stricter: since July 2018, all plants produced through genome editing methods in the European Union have been covered by the Genetic Engineering Act. The use of such plants, but also any release of the latter into the environment, must be first authorised, while food and feed produced from them must be labelled.
Voices on Genome Editing:
“Genome editing is an opportunity for democratisation, a very well-researched yet simple method that can be used to create innovative products without huge costs.”
Doctor Sarah M. Schmidt, scientist at the Department of Molecular Physiology at Heinrich Heine University Düsseldorf, and plant biologist Wolf B. Frommer, Humboldt Professor at Heinrich Heine University Düsseldorf in an article published by faz.de
“The focus (...) should be on the potential risk posed by a novel plant, regardless of the technology that was used to produce it. This approach would be in the interest of consumers and researchers alike, and it could open up new opportunities for a future-oriented, sustainable agriculture.”
Professor Dr Katja Becker, biochemist and molecular biologist at Giessen University and Vice President of the German Research Foundation (DFG), in a statement requested by the Science Media Center Germany (SMC).
“Genetic modifications achieved with the help of CRISPR/Cas are clearly to be classified as genetic engineering (...) Since genetic engineering legislation has entered into force, point mutations produced by means of traditional genetic engineering methods have been regarded as genetically modified organisms (...) This is so for a good reason. Even small interventions may lead to far-reaching changes in the properties of an organism and thus have relevant effects on humans and nature.”
Dr Margret Engelhard, head of the Department of Assessment of Genetically Modified Organisms / Genetic Engineering Act at the German Federal Agency for Nature Conservation (BfN), in a statement requested by the Science Media Center Germany (SMC).
How does genome editing with CRISPR-Cas9 work? This video by the Max Planck Society will show you: